На сегодняшний день большая часть добывающего фонда с ЭЦН оборудованна системой погружной телеметрии (ТМС), которая, в частности, включает в себя датчик давления на входе в насос.
Учитывая, что режимы скважин постоянно меняются в силу технологических обстоятельств, это делает возможным анализ реакции датчика давления ТМС с целью оценки интерференции с окружающими скважинами. Несмотря на видимую привлекательность, на этом пути есть серьезные практически ограничения: - Низкая чувствительность датчика давления ТМС.
Как правило чувствительность составляет 1 атм, и даже если возможность перевести его в режим 0.01 атм – это все-равно низкая чувствительность для амбициозных целей гидропрослушивания, к тому же исторические данные как правило записаны с чувствительностью 1 атм, даже если он поддерживает режим 0.01 атм – именно с этим приходиться иметь дело на практике.
- Грубый шаг записи датчика давления по времени.
Зачастую данные пишутся несколько раз в сутки, а часто и один раз в сутки.
- Плохой контроль за давлением на нагнетательных скважинах
Иногда нагнетательные скважины оборудованы устьевыми манометрами на линии (до штуцера) и в этом случае информативность по реакции на окружение очень низкая. В случае если нагнетательная скважина оборудована устьевым манометром на буфере (после штуцера), то у него есть шансы отреагировать на смену режимов окружающих скважин и применить МДКВ, хотя чувствительность такого датчика будет хуже, чем у погружного из-за демпфирующего влияния ствола.
Таким образом, наиболее подходящим методом анализа исторических данных по ТМС является радиальная деконволюция (РДКВ) вокруг добывающей скважины (по которой известна длительная история дебитов и забойного давления) и окружающих ее добывающих и нагнетательных скважин, от которых принимается во внимание история дебитов и расходов.
По итогам применения РДКВ к N скважинам получается N переходных характеристик (ПХ) : - Одна диагональная для центральной скважины ТМС, которая характеризует влияния смены режимов центральной скважины на ее собственное забойной давление
- N-1 кросс-диагональных ПХ в направлении окружающих ее скважин, которые характеризуют влияние смены режимов окружающих скважин на пластовое давление в центральной скважины
В силу низкого качества данных по давлению, качество ПХ будет тоже низким.
Очень часто это не позволяет анализировать ранние и средние времена диагонального элемента на предмет оценки влияния трещины, аккуратных оценок скин-фактора и гидропроводности.
Однако оценка пластового давления и поздних времен может оказаться весьма успешной.
Вот список параметров которые можно получить на основе РДКВ по типовым скважинам с ТМС:
- Пластовое давление по центральной скважине на произвольный момент времени (этот параметр устойчив к неточностям диффузионной модели)
- Сравнительный вклад окружающих скважин в динамику пластового давления центральной скважины (кто влияет на центральную скважину больше, а кто меньше)
- Качественная оценка динамики пластового давления в районе центральной скважины и ее окружения под влиянием внешних (не входящих в РДКВ тест) окружающих скважин (либо тенденция к истощению, либо тенденция к полной компенсации и выходу на стационар, либо отсутствие влияния внешнего окружения)
- Коррекция дебита центральной скважины (что может использоваться при реаллокации данных по ГЗУ)
Эта информация представляет собой большую ценность для оперативного анализа разработки, а также может использоваться для калибровки 3Д моделей. Необходимо помнить, что помимо качества исходной информации методы МДКВ имеют и естественные ограничения по информативности, в случае если режимы скважин менялись слабо и редко.
Пример Здесь напрашивается хороший пример по РДКВ на основе ТМС с добывающими и нагнетательными скважинами – бросьте картинки и я напишу текст. (https://www.arax.team/company/personal/user/20/tasks/task/view/8642/)
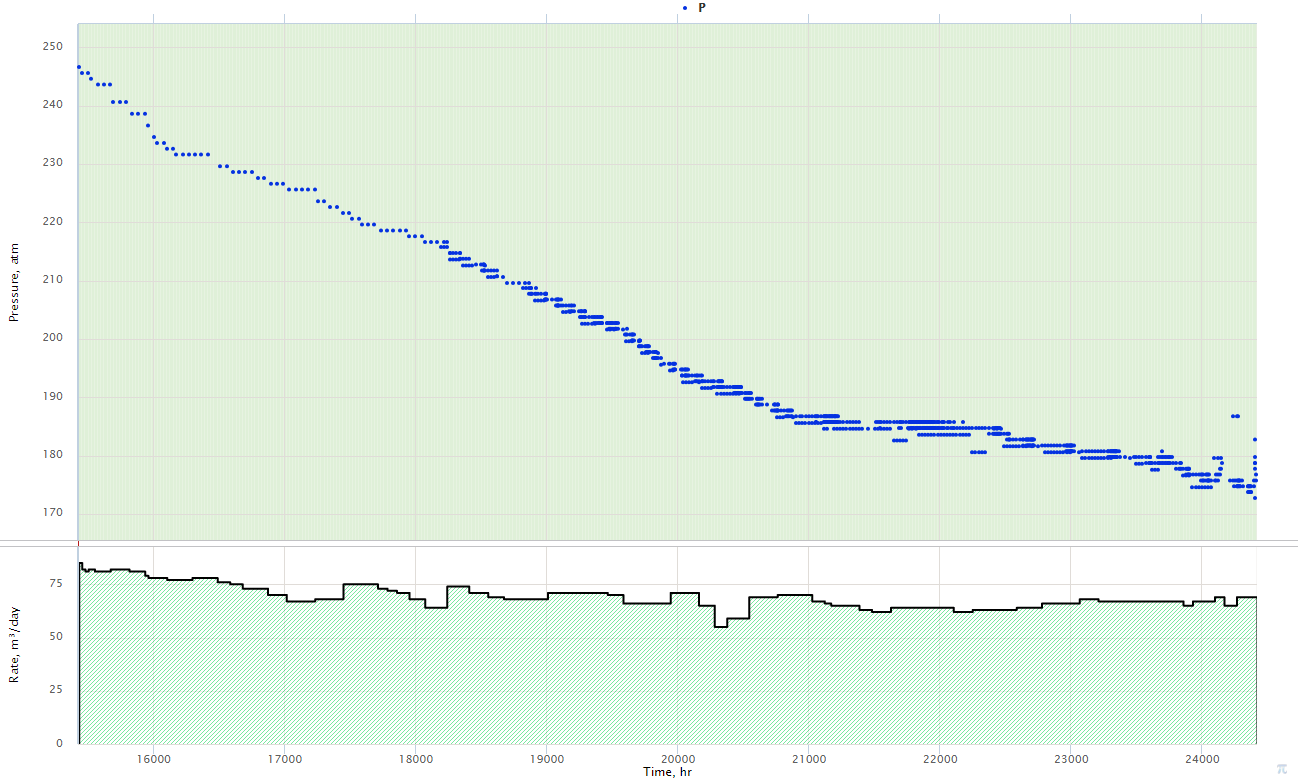
Рис.1. Запись давления ТМС и дебитов скважины OP-6 Должна быть картинка иллюстрирующая грубую запись давления ТМС, грубую запись нагнетательной скважины (https://www.arax.team/company/personal/user/20/tasks/task/view/8642/) 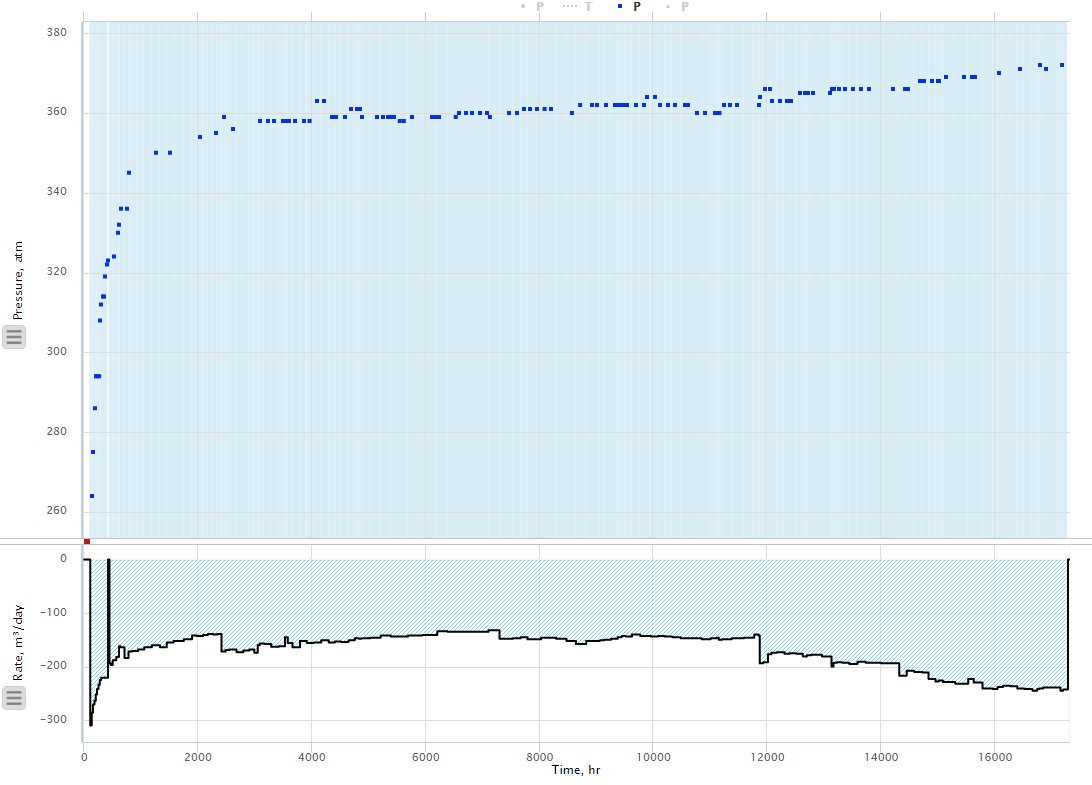
Рис.2. Запись давления и дебитов скважины Wl4 Карта с накопленными и текущими отборами (https://www.arax.team/company/personal/user/20/tasks/task/view/8642/)
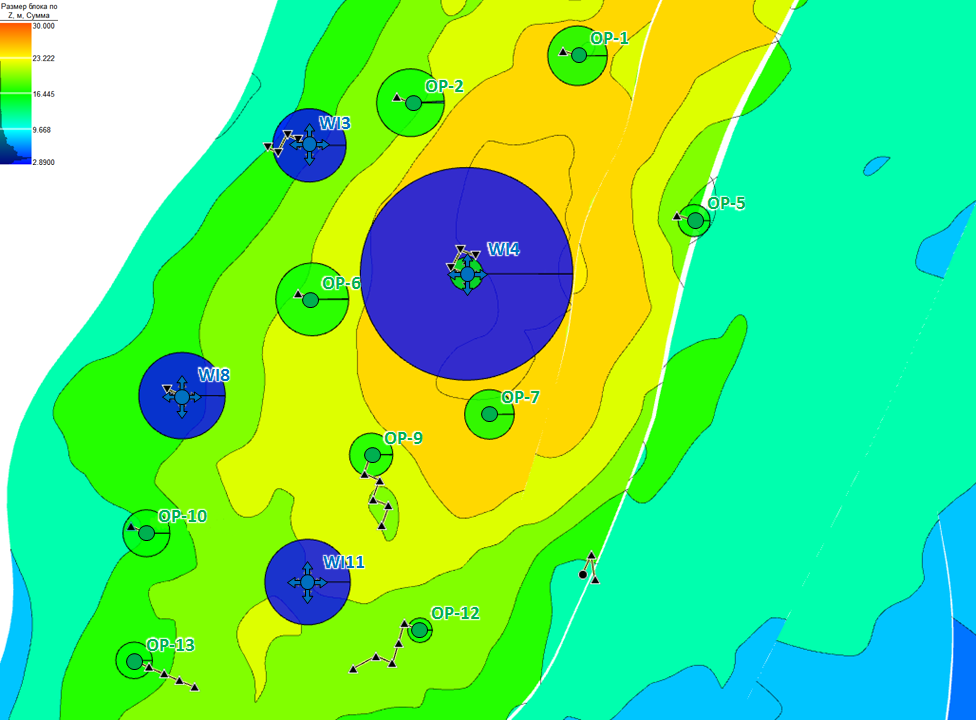 Рис.3. Карта накопленных отборов на 01.05.17 (подложка - карта толщин) 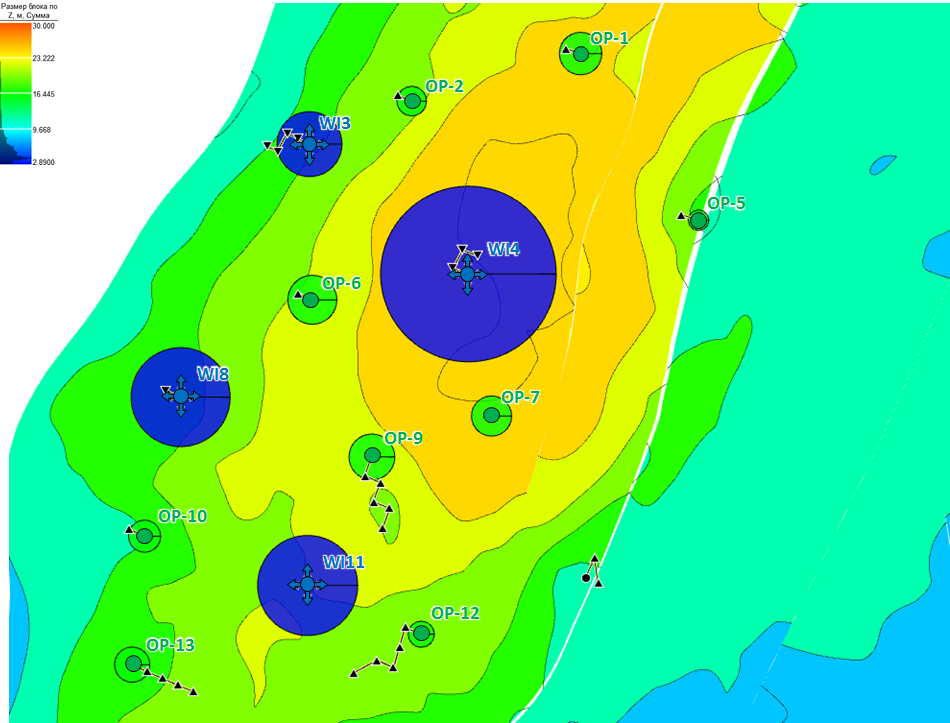
Рис.4. Карта текущих отборов на 01.05.17 (подложка - карта толщин) Портянка (https://www.arax.team/company/personal/user/20/tasks/task/view/8642/)
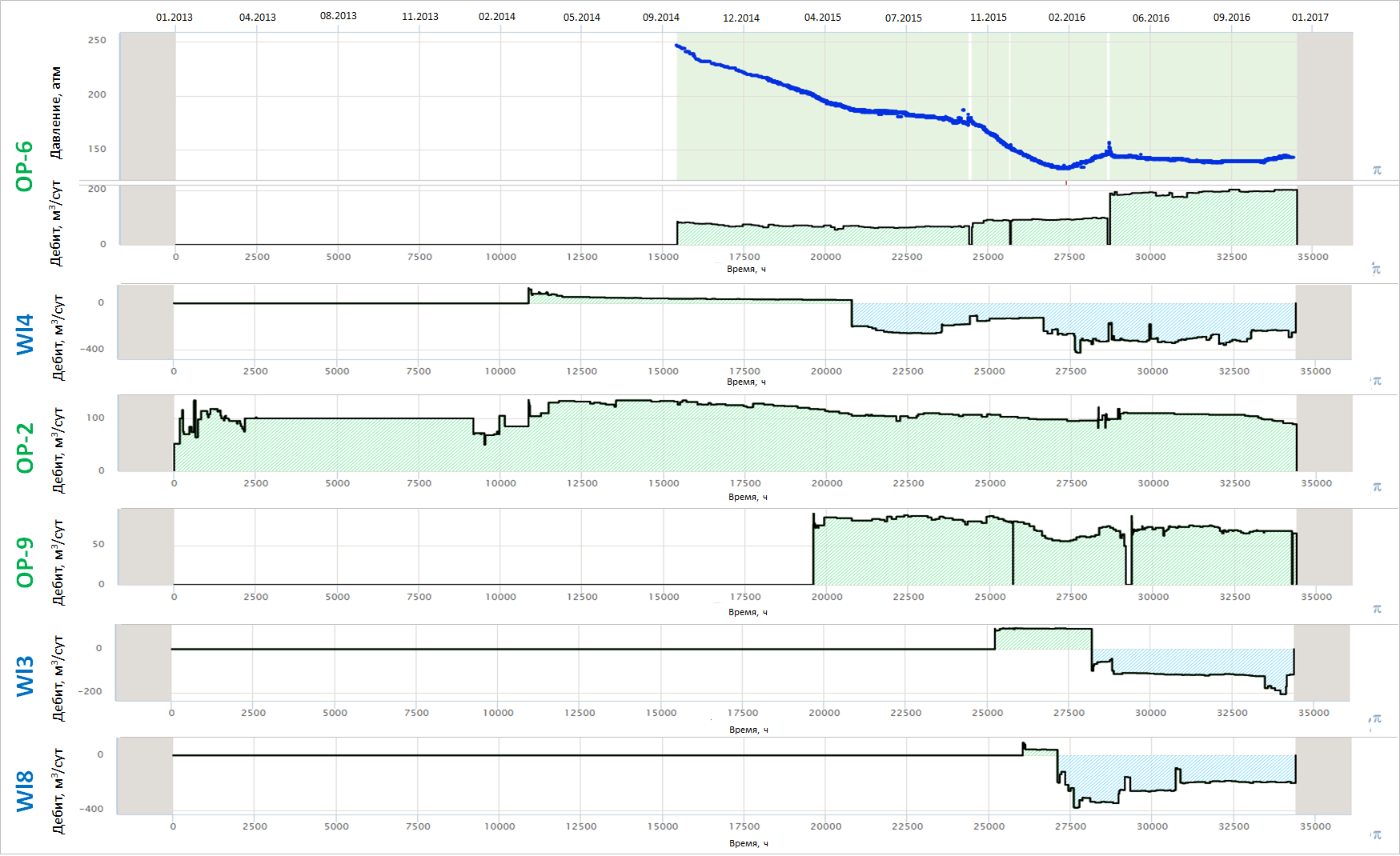 Рис.5. Дебиты и давления скважины OP-6 и дебиты окружающих скважин. ПХ (https://www.arax.team/company/personal/user/20/tasks/task/view/8642/) | Рис.6. Переходная Характеристика РДКВ OP-6 → OP-6 |
Динамика пластового давления центральной скважины (https://www.arax.team/company/personal/user/20/tasks/task/view/8642/)
| Рис. 7. Реконструкция пластового давления и депрессии на пласт в скважине OP-6 |
Сравнительный вклад окружающих скважин в пластовое давление по центральной скважине (https://www.arax.team/company/personal/user/20/tasks/task/view/8642/)
| | Рис. 8. График изменения пластового давления в зависимости от влияния соседних скважин на OP-6 | Рис. 9. График изменения пластового давления в зависимости от влияния соседних скважин на OP-6 (зум) |
Коррекция дебитов по центральной скважине – зум вокруг явной ошиьки в исторической записи (https://www.arax.team/company/personal/user/20/tasks/task/view/8642/) 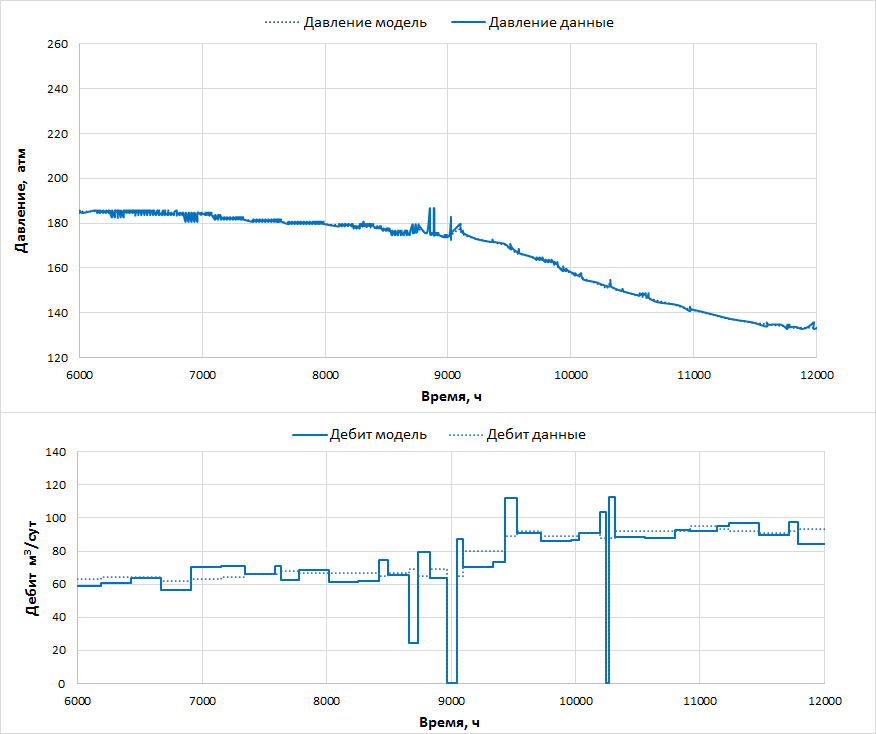 Рис. 9. График коррекции дебитов и давлений по РДКВ скважины OP-6
|